Drug-eluting stents were a breakthrough in preventing restenosis after angioplasty and stent implantation. By slowly releasing drugs that inhibit smooth muscle cell proliferation, they reduced restenosis rates compared to bare metal stents. However, drug-eluting stents only provide a temporary solution. Once the drugs are exhausted, the stents behave like traditional stents and restenosis risk increases again. With the growing use of stents, even a small risk of complications translates to large numbers of in-stent restenosis and thrombosis cases that require repeat procedures or lead to morbidity and mortality.
Permanent solutions are needed to overcome the limitations of drug-eluting stents. These could include biodegradable stents that gradually dissolve in the body after providing temporary support, eliminating risks from permanent implants. Their degradation rate must be carefully controlled to match the healing response. Bioactive surface coatings are another option, incorporating materials that actively promote endothelial cell growth to accelerate healing and embed the stent in the vessel wall.
Biocompatible surfaces that prevent protein and cell adhesion could also help resolve inflammation and thrombosis risks. Certain nano- or micro-scale surface topographies have shown promise inhibiting smooth muscle cell proliferation while supporting endothelial cell growth. Permanent polymers, metals or ceramics with optimized properties could potentially serve as non-degradable coatings to avoid risks from permanent stents if the surface prevents adhesion.
The ideal solution may incorporate multiple approaches, e.g. biodegradable stents with bioactive coatings and non-thrombogenic surfaces. A multi-disciplinary approach combining biomaterials, drug delivery, surface engineering and medical expertise will be required to develop permanent treatments for in-stent restenosis and thrombosis. With further progress, permanent stent solutions could eliminate the need for repeat procedures, improving outcomes and quality of life for patients requiring stents. The ability to apply a stent then avoid repeat interventions would provide benefits beyond reducing health care costs alone.
Overall, we have made great progress in preventing restenosis with drug-eluting stents but permanent solutions remain elusive. Eliminating risks from permanent implants while optimizing biocompatibility and the healing response will require scientists and clinicians working together to develop the next generation of smart stent solutions. But the rewards of achieving a single, permanent solution to in-stent restenosis would be well worth the effort. Patients rightfully expect more from 21st century medical technologies.

Conventional Solutions Only Provide Temporary Relief
Drug-eluting stents incorporate drugs in a polymer coating that slowly release over time to prevent smooth muscle cell proliferation and restenosis. While effective at reducing restenosis rates compared to bare metal stents for a limited time, drug-eluting stents only offer a temporary solution. Once the drug supply is depleted, the stents behave like traditional stents again and restenosis risks return. With the increasing use of stents, even small complication rates translate to large absolute numbers of in-stent restenosis and thrombosis cases requiring repeat interventions.
Drug supplementation is another conventional approach, administering anti-platelet or anti-proliferative drugs to patients after stent implantation to inhibit cell proliferation and reduce clotting risks. However, drug treatments also only provide temporary effects and can lead to problems with patient compliance or side effects over long-term use. Systemic drug delivery may also impact parts of the body beyond the stented arteries.
Bioabsorbable stents were developed to provide temporary support to the vessel before degrading within 2-3 years. They were designed to reduce risks from permanent implants after the vessel heals. However, controlling degradation to precisely match the healing timeline in each patient remains challenging. Premature degradation or incomplete healing could lead to restenosis, while delayed healing risks in-stent thrombosis, similar to permanent stents. Outcomes have been mixed, showing no clear benefits of bioabsorbable stents compared to permanent stents thus far in clinical trials.
In summary, while conventional solutions like drug-eluting stents, drug therapy and bioabsorbable stents have significantly improved stent therapy by delaying or preventing restenosis and thrombosis for a limited time, permanent options are still needed to avoid repeat interventions and eliminate risks from permanent implants in the long run. A perfect solution continues to remain elusive, highlighting the complex challenges in developing a smart stent able to prevent restenosis and thrombosis permanently while avoiding other complications – but permanent answers could improve health outcomes for millions of patients requiring stents.
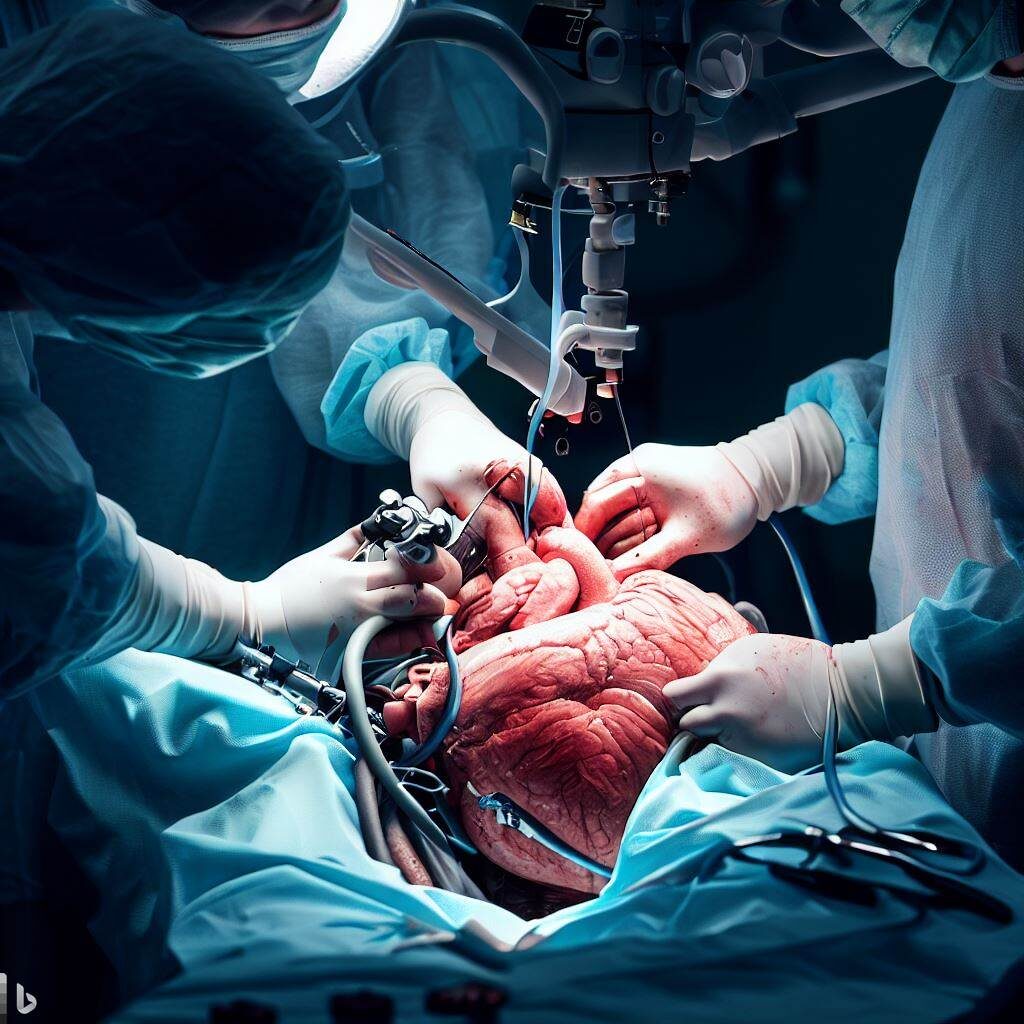
The Limitations of Drug Therapy for Stents
While drug-eluting stents have succeeded in reducing in-stent restenosis rates, drug therapy as a systemic approach also has significant disadvantages. Patients require extended anti-platelet drug regimens after stent implantation to reduce thrombosis risk. Long-term drug use can lead to issues with patient compliance, forgetting or choosing to stop medication, which increases the risk of stent thrombosis. Anti-proliferative drugs in particular can also cause side effects that reduce quality of life when taken over long periods.
Systemic drug delivery impacts parts of the body beyond just the stented arteries. This can cause complications in other parts of the circulatory system or non-targeted tissues affected by the drugs. Drugs that prevent smooth muscle cell proliferation and cell migration may also impact endothelialization of the stent, which is required for healing. Impaired healing increases the risks of both restenosis and stent thrombosis.
Drug resistance is another concern with long-term anti-platelet and anti-proliferative drug regimens. Over time, the body can become resistant to the effects of certain drugs, reducing their efficacy and requiring dose increases or changes in medication. However, too high drug doses can lead to greater side effects. Balancing drug dosage to avoid these side effects while maintaining therapeutic effectiveness remains a challenge and concern, especially for anti-proliferative drugs.
In summary, while drug therapy has improved outcomes for stent patients, systemic drug delivery is not a perfect or permanent solution either. Compliance issues with long-term drug regimens, side effects that reduce quality of life, impaired healing, and risks of drug resistance highlight the need for localized solutions able to prevent restenosis and thrombosis through surface properties or mechanical factors rather than relying exclusively on drug release. Combining temporary drug therapy with permanent design solutions could provide benefits over either approach alone. As with any treatment, a balanced, multidisciplinary approach is needed to maximize therapeutic effects while minimizing risks to the patient.
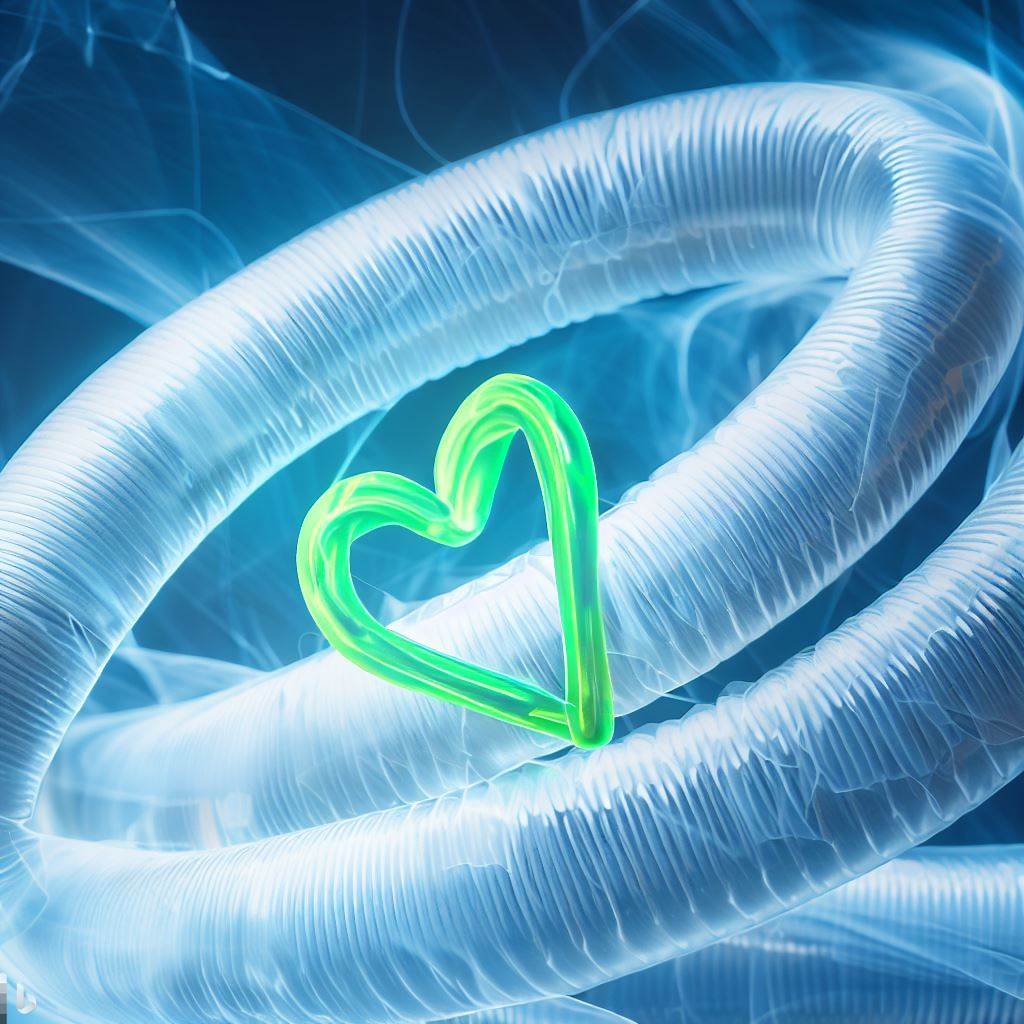
Promising Solution Strategies for Future Work
Biodegradable stents provide temporary support before gradually dissolving completely within the body. Matching their degradation rate to the healing timeline could eliminate risks from permanent implants after serving their purpose. However, precisely controlling degradation in each patient to optimize healing remains challenging. Novel materials and designs will be needed to ensure adequate strength and support during healing while enabling programmed dissolution afterwards. More work is required to develop biodegradable stents providing benefits over permanent stents.
Bioactive and biocompatible coatings incorporate materials to actively promote healing or passively prevent adverse responses like thrombosis. Bioactive coatings containing growth factors or peptides could stimulate endothelial cell attachment and proliferation to speed healing and embed the stent in the vessel wall. Non-thrombogenic coatings prevent protein and platelet adhesion to avoid short- and long-term thrombosis risks from permanent implants. Surface coatings may provide more localized effects without systemic side effects, if developed and applied appropriately for stents.
Optimizing stent geometry, material selection and mechanical properties offers another approach to improving compatibility and long-term performance. Stent designs enhancing endothelial coverage and smooth muscle cell inhibition show promise. Materials like magnesium alloys with tailored corrosion resistance and strength degradation profiles could provide stents suitable for temporary implantation. Surface micro- and nano-scale features may also discourage smooth muscle cell proliferation while encouraging endothelial cell growth for faster healing, if properly designed and manufactured. More work is still needed to optimize designs for safety and efficacy.
In summary, biodegradable stents, bioactive and biocompatible coatings, and optimizing stent designs based on material selection and geometry show promise for improving stent compatibility and long-term outcomes. However, translating these strategies from concepts to clinical therapies will require significant research and development. Precisely controlling biodegradable stent degradation, ensuring the safety and efficacy of surface coatings, and optimizing stent designs for maximum therapeutic potential while avoiding complications remain complex challenges necessitating a multidisciplinary approach. With further progress, these solution strategies could provide the next generation of smart stents to make repeat interventions a thing of the past.
Polymer Stents: A Promising Solution Strategy
Biodegradable polymer stents provide temporary scaffolding to support the vessel after implantation before gradually degrading within the body. Matching the degradation rate to the healing process could eliminate risks from permanent implants after they have served their purpose. Polymers like poly-L-lactic acid (PLLA), polycaprolactone (PCL) and polyvalerate (PVA) have been investigated for biodegradable stents due to their biocompatibility, tunable degradation properties and mechanical strength.
However, polymer stents also have significant disadvantages to overcome before clinical use. Ensuring polymer stents have adequate strength and support to prevent restenosis during the critical healing period remains challenging. Premature degradation could cause restenosis before the vessel has healed. Polymers may produce acidic degradation products that cause inflammation if not properly designed. Controlling degradation precisely in each patient is difficult. While polymer stents are promising in theory, they currently lack sufficient performance and safety for most applications.
More work is needed to develop optimized polymer materials and stent designs that provide targeted strength and degradation. Material selection, processing techniques and geometry should be tailored to specific applications and timelines. Combining polymer stents with other strategies like bioactive coatings may address their limitations by promoting healing while the stent degrades. Manufacturing optimizations can enhance polymer stent properties towards overcoming disadvantages like limited strength before degradation and unfavorable degradation byproducts.
With continued progress in material selection, optimal design and combination treatments, biodegradable polymer stents could provide temporary support for the vessel until healing is complete, eliminating risks from permanent implants. However, translating this promising concept into a clinical reality will require interdisciplinary expertise focused on meeting the complex and demanding design requirements for this application. Polymer stents are still in their infancy, highlighting the significant work ahead to develop them as a viable solution for in-stent restenosis. But their potential advantages warrant the effort to systematically address remaining challenges through further research.

Polymer-Coated Biodegradable Metal Stents: The Best of Both Approaches?
Polymer-Coated Biodegradable Metal Stents: The Best of Both Approaches?
Biodegradable metal stents provide temporary scaffolding before corroding away within the body at a controlled rate. Magnesium alloys are popular for biodegradable stents due to their intrinsic biocompatibility, suitable mechanical properties and corrosion profiles. However, their degradation also releases hydrogen gas, increases pH and forms oxide/hydroxide products that can delay healing. Polymer coatings may address these effects when applied to magnesium stents.
Polymers can act as barriers between the metal stent and surrounding tissues, controlling degradation products from reaching the vessel walls. They also enable localized drug delivery to prevent restenosis while the stent degrades. A polymer coating tailored to degrade at the same rate as its magnesium substrate could provide an optimized solution. For example, PLLA or PCL coatings have shown promise controlling magnesium degradation and restenosis when applied to stents. The polymer controls byproducts and release of physically absorbed or encapsulated drugs until it clears from the stent surface, by which time the vessel has ideally healed sufficiently.
Polymer-coated biodegradable metal stents aim to combine the benefits of polymer and metal approaches, enabling programmed biodegradation of the entire device with controlled drug release to prevent restenosis during the critical healing window. However, optimizing polymer coatings to achieve safe, targeted and efficacious performance on biodegradable stents remains challenging, especially matching coating degradation to the complex in vivo corrosion of the metal. Drug choice, dose and release kinetics also require optimization for specific applications. Precision manufacturing processes are needed to apply uniform polymer and drug coatings to stents.
While promising, polymer-coated biodegradable metal stents still require substantial research and development before clinical use. Ensuring their performance, safety, biocompatibility and corrosion resistance for the intended application will demand an interdisciplinary approach focused on balancing requirements for both the polymer coating and biodegradable metal. When and if these complex challenges can be addressed, combining polymer coatings with biodegradable stents could provide an innovative solution enabling temporary scaffolding and drug delivery, followed by programmed elimination of the entire device to avoid long-term risks. The potential advantages are exciting but first translating this concept into reality will require significant scientific progress through further study.
Polysaccharide Coatings for Biodegradable Metal Stents
Polysaccharides are complex carbohydrates with intrinsic biocompatibility and biodegradability that can be used to coat biodegradable metal stents. Their natural origin may avoid undesirable inflammation caused by certain synthetic polymers. Polysaccharides like alginate, chitosan and hyaluronic acid provide temporary barriers between the stent and surrounding tissues, controlling byproducts from corroding metals like magnesium by delaying their release and transport. They can also be used as a matrix for drug release to inhibit restenosis while the stent degrades.

However, polysaccharide coatings present significant challenges for stent applications. Achieving uniform coatings with targeted thicknesses requires precision manufacturing processes that avoid delamination or cracking on stent expansion. Ensuring adequate adhesion and distribution of polysaccharide and any incorporated drugs to treat the entire stented area demands tightly controlled coating procedures. Matching the degradation of polysaccharide coatings to that of the metal stent in vivo is complex, and prior to clearing from the stent their breakdown products must avoid issues like inflammation or fibrous encapsulation. Approaches to mitigate hydrogen gas production or modulate magnesium ion concentrations, for example, may still be needed with polysaccharide-coated stents.
Polysaccharide-coated biodegradable stents aim to utilize natural materials to control metal degradation processes and enable drug delivery until natural breaking down and removal of both coating and stent from the body. In theory, this temporary stent system could provide essential scaffolding and treatment during the crucial initial healing period before dissolving away, avoiding risks associated with permanent implants. However, transitioning polysaccharide-coated biodegradable stents from concept to clinical reality will require considerable research to determine optimal coating materials, uniform deposition techniques, drug choices, dosage and release profiles for safe and effective performance, and balancing with stent metal selection and geometry.
Significant scientific challenges involved in developing polysaccharide-coated biodegradable stents highlight the complexity of this promising solution strategy. When and if these challenges are addressed through interdisciplinary collaboration, targeted stent coatings utilizing naturally derived polysaccharides could provide an innovative approach enabling temporary support and localized drug therapy paired with programmed elimination of the entire device. But realizing the advantages in practice will first demand a systematic, application-focused research effort to meet the demanding requirements involved in designing temporary, fully biodegradable stent-coating systems suitable for evaluation beyond basic proofs-of-concept.
Bio-Derived Polymers for Biodegradable Stents
Polydopamine is a biocompatible polymer that can spontaneously coat metal surfaces, promoting cell adhesion and growth. Used on biodegradable Mg stents, polydopamine coatings accelerated endothelialization while reducing inflammation and restenosis in animal studies. They may control byproducts from Mg degradation like hydrogen gas release. Other bio-derived polymers include silk, fibrin and tropoelastin – natural materials that support cell growth and provide mechanical integrity when processed into coatings or porous foams. On biodegradable stents, these materials could enhance healing while the stent degrades then break down themselves.
Bio-derived polymer coatings may provide benefits over synthetic permanent polymers, but optimizing them for stents remains challenging and requires extensive research. Uniformly coating stents with controllable thicknesses demands specialized techniques. Achieving safe, targeted performance will necessitate determining choices and doses for any incorporated drugs, profiles for their release, and matching coating degradation to the complex in vivo corrosion of metals like Mg. Effectively tying together natural materials with complex chemical/physical properties to prevent inflammation or fibrous encapsulation before elimination from the body must be demonstrated.
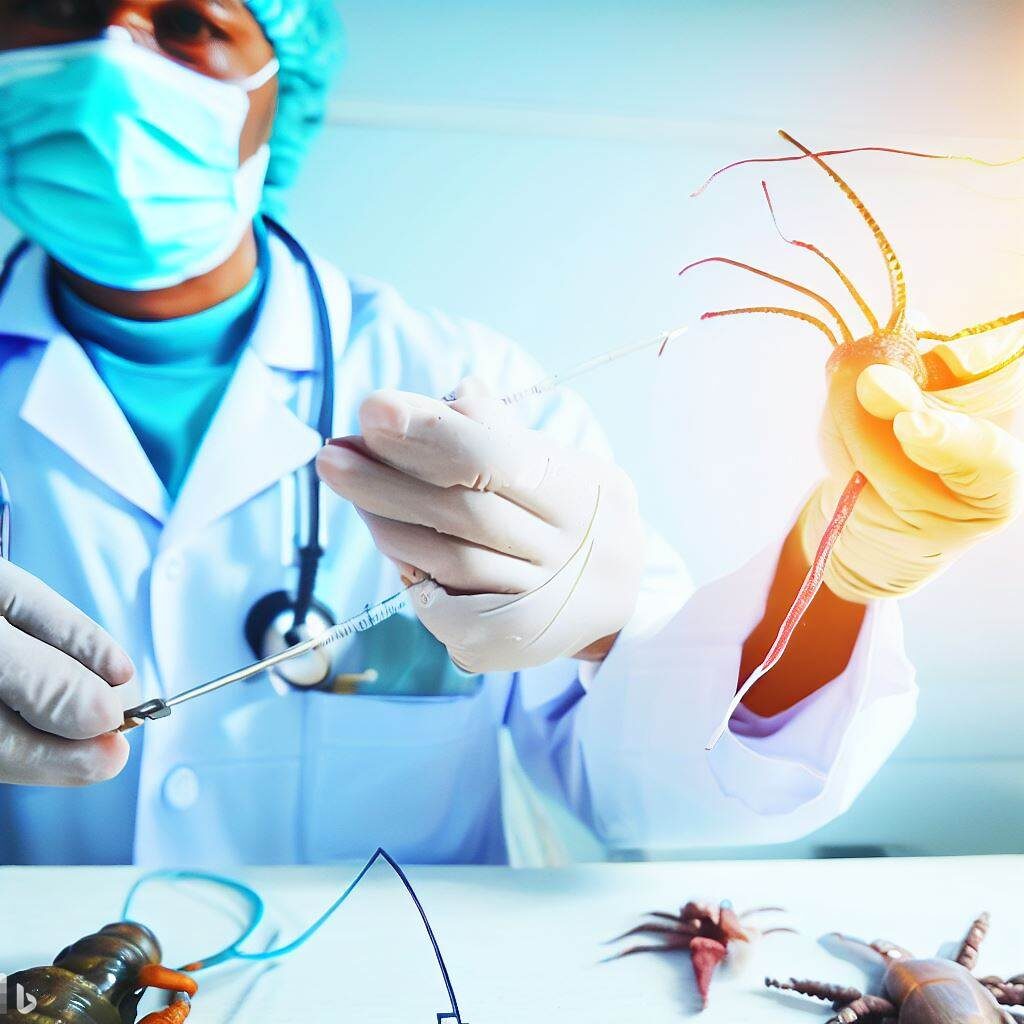
Coatings utilizing natural materials aim for an innovative solution enabling temporary scaffolding, enhanced healing, programmed degradation and removal of polymer and metal from the body. However, this promising concept still faces practical roadblocks in systematically developing bio-derived polymer coatings suitable for temporary fully biodegradable stents. The level of research necessary includes:
- Optimizing materials, processing and designs for targeted applications, balancing synergistic effects.
- Defining performance, safety and biocompatibility requirements to achieve controlled degradation synchronized with natural healing.
- Carefully evaluating drug and release kinetics choices to maximize benefits.
- Applying precision deposition methods to obtain uniform coatings on stents before expansion.
- Assessing outcomes beyond basic proofs-of-concept through in vitro and in vivo studies.
While appealing in theory to avoid permanent implants, natural polymer-coated biodegradable stents require substantial scientific progress through interdisciplinary efforts focused on meeting complex demands. When and if these are addressed, polydopamine, silk, fibrin, tropoelastin and other bio-derived materials have exciting potential for innovative temporary stent coatings followed by full biodegradation. But transitioning from promises to reality will demand analysis, planning and development systematically confronting obstacles of processing highly chemically/morphologically variable natural polymers for medical devices. With adequate research, these coatings could eliminate needs for surgical removal by seamlessly breaking down after their therapeutic window closes – but only diligent work will determine if their possibilities outweigh practical challenges enough for clinical translation.
An Optimized Solution: Merging Approaches for the Ideal Biodegradable Stent
Combining multiple promising strategies could provide an optimized biodegradable stent solution. For example, a magnesium alloy stent coated with a bio-derived polymer like polydopamine, incorporating an anti-proliferative drug with its release profile tailored for the critical healing window. The polydopamine enhances healing and controls Mg degradation until clearing from the stent. The drug prevents restenosis while the stent provides temporary support before degradation.
Once the polymer coating and drug deplete after ~3-6 months, the bare Mg stent would continue controlled degradation over a period tuned to when natural vessel strength returns, then fully resorb within 12-24 months. This approach utilizes the best of both metal and polymer techniques while maximizing efficacy and minimizing side effects. Tailoring and synchronizing components to temporary requirements could achieve a “smart” biodegradable stent solution enabling essential scaffolding with temporary localized drug delivery, followed by natural healing and total removal of the implant from the body.
A synergistic combination of optimized biodegradable stent platform (magnesium alloy), bio-friendly surface modification (polydopamine) and drug regimen ensures 1) sufficient strength during healing, 2) controlled degradation and byproduct release (via coating), 3) minimized restenosis risk (via drug release profile), and 4) seamless transition to natural vessel recovery (as components disappear). IVUS or similar monitoring may be used to guide design and performance specs for specific patient needs. The end result: a temporary stent system providing therapy then naturally breaking down and safely passing from the body without surgical intervention.
Challenges demand that all elements of this approach be systematically developed and tuned together for maximum efficacy and safety. But a device enabling targeted support through the most critical phases of healing and risk, followed by removal of all traces after serving its purpose, would provide clinical advantages for many applications. With customized components and properties for different needs, optimized biodegradable stents merging metals, bio-coatings and drugs could eliminate risks from permanent implants after stabilizing and healing vessels. The ideal solution may be a synergistic one.

The Promise and Challenges of Biodegradable Stents: Where We Are and Where We Aim to Be
Significant progress has been made developing biodegradable stents as alternatives to permanent implants. Materials like magnesium and bio-derived polymers show promise for stents providing temporary support before disappearing naturally from the body. Coatings and drug regimens may enhance their performance. However, optimizing biodegradable stents has proven complex, demanding synchronized control of strength, degradation and healing for safe and efficacious treatment. Restenosis and thrombosis risks must be minimized through the critical period as structures deteriorate and byproducts arise, then clear uneventfully.
While polymers, magnesium and surface coatings are appealing in theory to avoid permanent implants, they require substantial work for clinical reality. Determining optimal materials, designs and processing; defining performance requirements for applications; ensuring safety and efficacy, especially with degradation; synchronizing temporary components; and evaluating outcomes remain challenging, necessitating significant research. Bio-derived polymers and natural materials also face chemical/physical variability introducing complications not seen in synthetics. Drug choices and tailored release profiles are needed for anti-thrombotic and anti-proliferative effects during the high-risk window as temporary structures degrade.
With progress meeting current challenges, biodegradable stents could provide a breakthrough solution. Fully customized systems with stents, coatings and drug formulations tuned to specific needs may be possible. A synergistic approach optimizing multiple strategies could enable stents with temporary drugs and enhanced healing, seamlessly passing from the body once natural recovery takes over – a “smart” implant providing targeted support through critical periods then auto-eliminating with a built-in self-destruct mechanism, visible or MRI monitoring may guide performance needs. The possibilities of temporary biodegradable combination devices with designed control and site-specific therapy are exciting if we systematically determine how best to achieve it.
Interdisciplinary teams focused on balancing complex practical challenges with promising options for temporary implants that avoid complications of permanent devices have the opportunity to develop biodegradable stents achieving the high ideals imagined when first conceived. But we must walk before running, building this vision piece by piece through diligent material, processing and translational analysis confronting obstacles, not just touting theoretical advantages. With work, biodegradable stents might reach their potential enabling stabilizing treatment through vulnerable periods then stepping away naturally when their job is done – a futuristic solution only made possible by aggressively tackling limitations of the present. The future remains unwritten, but through concerted effort we can determine if it includes temporary implants providing therapy then disappearing on cue without a trace.